Recently, the research team at NEU focusing on external field control in metallurgy and materials processes published a research paper titled "Magnetic field assisted synthesis of dense braided rope NiCoP as a highly efficient hydrogen evolution electrocatalyst" in the prestigious international journal Applied Catalysis B: Environmental. NEU is the first author institution, with Wang Zhong, a doctoral student from the School of Metallurgy at NEU, as the first author. Professors Wang Qiang and Associate Professor Yuan Shuang are the co-authors.
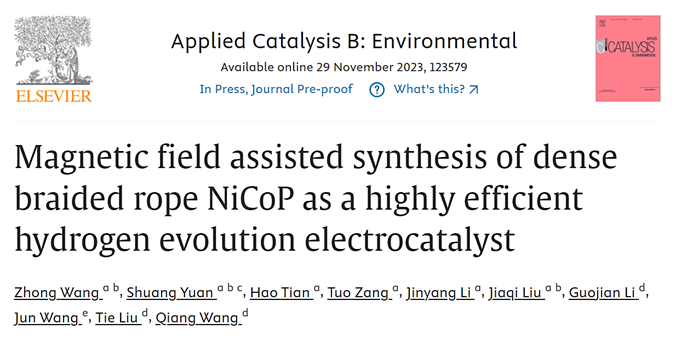
Under the current circumstance of growing energy demands and the depletion of fossil fuels threatening sustainable development, the development of clean energy has become an urgent task for replacing fossil fuels and promoting a low-carbon or zero-carbon economy. Hydrogen energy, with its high energy density and no carbon emissions during use, holds great promise for various applications, especially in fields such as metallurgy.
Renewable energy-driven electrocatalytic water splitting for hydrogen production is considered the most promising green hydrogen production method and an important energy storage approach to address the unreliability and intermittency of renewable energy sources. However, the widespread application of electrocatalytic water splitting is hindered by the high cost and scarcity of precious metal catalysts. To achieve the national "dual carbon" strategy and advance the hydrogen energy industry beyond energy challenges, the development of low-cost and abundant non-precious metal catalysts is of great significance.
The research team at NEU's Metallurgy and Materials Process External Field Control employed magnetic field regulation to control the growth process of catalyst materials, overcoming various limitations in traditional preparation methods. They achieved the synchronous regulation and efficient preparation of highly active NiCoP catalysts in terms of morphology, elemental ratio, and capacity. Combining COMSOL numerical simulation and high-speed camera observation, they clarified the effects of the magnetic field on solvent concentration polarization, Ni/Co load ratio, gas evolution, and detachment processes, revealing the impact mechanism of the magnetic field's magnetohydrodynamic effects on the aforementioned processes. The team also used Density Functional Theory (DFT) to uncover the effects and mechanisms of different Ni and Co metal element contents on catalyst performance.
To explore the possibility of catalyst scaling and commercialization, the team assembled a "solar panel-electrolyzer-fuel cell" system using the catalyst. This system utilized solar panels to drive electrocatalytic water splitting for hydrogen production and subsequently employed hydrogen gas to power a fuel-cell vehicle. Through experimental tests, the team validated the high activity and efficient hydrogen production capabilities of the NiCoP catalyst prepared under a magnetic field.
The study demonstrated that the magnetic field effectively regulated the morphology of NiCoP catalysts. Compared to nanoflower-shaped NiCoP prepared without a magnetic field, NiCoP-600 showed a larger electrochemical active surface area, faster electron transfer capability, and better hydrophilic performance. The magnetic field also optimized the ratio of Ni to Co and the yield of the catalyst, contributing to the optimization of catalyst performance.
In addition to this work, the team has explored various aspects of catalyst structure regulation and optimization under a magnetic field in the past two years, and they have achieved brilliant research results. The related technologies will provide theoretical and technical support for the future design and preparation of highly active catalysts and other functional materials.